Water pollution! Mycelium solution?
Laboratory experiments to test the ability of fungi mycelium to reduce the concentrations of various contaminant substances from agricultural runoff water and/or effluent.
Alexander M. Olieman and Maurice Veerkamp
Summary
Water pollution due to agricultural runoff is a problem that society is trying to address in various ways. As fungi play an important role in soil ecology and especially in nutrient cycles, they facilitate a broad range of metabolic processes. This behavior might enable them to remove excess nutrients and other compounds from agricultural runoff, by acting as living filters. The proposal will focus on these beneficial effects that particular species of fungi can have in removing contaminants from polluted water.The research will take place over a four year period in during which the research will look at the specific species of fungi and their correlation to water pollution in the ‘wild’. The fungi will go through a process of strain isolation to select the ones with the best growth potential. After having obtained these strains, they will be tested to identify those which are likely to perform well under realistic conditions. The last experiment of the research proposal approximates realistic conditions and tests the selected strains for their ability to decrease concentrations of water contaminants.
This research will give insight into which fungi species to select or ‘train’ (strains thereof) to be applied into mycofilters, usable in areas in the Netherlands where issues with agricultural contaminants have been reported.
Research Question
The following research questions are situated within the field of mycoremediation; an inquiry into the ability of fungi to degrade or accumulate contaminating substances in an environment. Research that is conducted in the field of mycoremediation makes use of the central disciplines biology, chemistry, ecology, and economics.Main research question
Which fungi species can reduce the concentration of agricultural contaminants in water, in a laboratory setting that approximates realistic conditions?
Phase 1
Which fungi species that can reduce agricultural contaminants can be found in literature? Taking the genetic diversity of fungi into account, how large should sample sizes be in order to make reliable statements about which species show promise? Which contaminants of agricultural origin cause most societal/economic problems in the Netherlands?
Phase 2
Is there a significant correlation between the occurrence of specific species and specific contaminants?
Phase 4
What is the influence of contaminant(s) x1(, x2, x3,...xn) with concentration(s) y1(,y2,...yn) on the growth of strain z.
Phase 5
What is the uptake/breakdown capability of contaminant(s) x1(, x2, x3,...xn) of different strains of (saphrophytic) fungi? Which strains of (saphrophytic) fungi show a high potential to be applied in mycofilters which can be used to reduce ground- and surface-water contamination from agriculture in the Netherlands?
Objective
Main objectiveTo identify species of (saphrophytic) fungi that show high potential for application in mycofilters which can be used to reduce ground- and surface-water contamination from agriculture in the Netherlands.
Phase 1
To identify fungi species that have already shown the ability to reduce agricultural contaminants in experimental settings. To gain knowledge on genetic diversity in fungi. To produce a ranking of which agricultural contaminants cause most societal/economic problems in the Netherlands.
Phase 2
Collecting a large number of mushroom and water samples at modest cost. Checking for significant correlation between the occurrence of specific species and specific contaminants.
Phase 3
To isolate strains from tissue samples that show the best growth potential on clean agar substrate. Idem, but with contaminated agar substrate.
Phase 4
Perform a series of agar well bioassays in order to identify strains that are likely to perform well under realistic conditions.
Phase 5
To measure the change in concentration of water contaminants after the solution has been led through a substrate that has been colonised by a fungus strain. To gain knowledge of the uptake/breakdown capability of contaminant(s) x1(, x2, x3,...xn) of the selected strains. To select or ‘train’ a strain of fungi to be applied into mycofilters, usable in areas in the Netherlands where issues with agricultural contaminants have been reported.
Theory
Fungi play an important role in soil ecology and especially in nutrient cycles. Because fungi facilitate such a broad range of metabolic processes, they might also be able to remove excess nutrients (or other chemicals) from an environment. Stamets (2005) has brought the importance of fungi in environmental remediation to the attention of large crowds. One example of a new application that he has found for fungal mycelium is the use of mycofilters around farms, watersheds, urban and industrial areas. These living membranes are found to filter pathogens, silt, organic debris, and chemical toxins from water runoff.Mycofiltration is a novel idea within the accepted method of mycoremediation (our theoretical point-of-departure); the use of fungi to degrade or accumulate contaminating substances in an environment. Suitable approaches for the in-situ use of mycoremediation are found in Stamets (2005), while Singh (2008) focuses on fungal-technological systems (bioreactors) to degrade contaminants. Jones (2009) has conducted a literature review of in-situ remediation uses of mycorrhizal fungi, but such a review is lacking for saprophytic fungi.
The application of mycofiltration to water pollution problems in the Netherlands seems promising when the possibilities for this invention are considered. De Vries (2009) has conducted a set of field trials and experiments, after which she concluded that "increased fungal biomass is [...] a cause of reduced nitrogen losses to the environment." Her results have shown that both mycorrhizal and saprophytic fungi contribute to this process. It is also fairly certain that fungi can assist in removing metals and aromatic hydrocarbons from contaminated soil (Elekes and Busuioc, 2010; Ermanno et al. 2007) and can remove metals from wastewater (Price et al., 2001). Mycofiltration patents have also been granted with the claims of addressing a large variety of organic compounds (Berthon and Grizard, 2004), and of removing toxins, metals, bacteria, viruses, petroleum products, pesticides, and fertilizers from water runoff (Stamets, 2003).
During the previous decade, scientific interest in contaminants from agricultural origins in our target region has mainly been in fertilizers/nutrients and pesticides (Maanen et al., 2000; Wolf et al., 2003; Schroder, 2004; De Zwart, 2005). We additionally predict that recent issues with E. Coli contamination of food products in Europe will increase the time spent on addressing xenobiotic contaminants from agriculture.
There are however currently too many unknown factors to justify a field trial with mycofiltration methods with the aim of addressing contaminants of farm runoff in the North Sea drainage basins. It is suggested (Stamets, 2005; Jones, 2009) that mycofilters are based on indigenous fungi species, so that the ecological effects of intervening in the farmland can still be overseen. There is however only little literature on mycofiltration experiments performed with species that are indigenous to Western Europe. Additionally, the specific make-up of contaminants from farm runoff and/or effluent does not allow us to predict the effectiveness of indigenous mycofilters from the available theory. Singh (2008) addresses the applications of mycoremediation to decompose synthetic pesticides, and uptake (fix) common nutrients, such as nitrogen and phosphorous, and heavy metals. Stamets (2005) also acknowledges these possibilities, and adds to this the suggestion that the enzymes and antibiotics which are secreted by mycelium can neutralize the threat of many pathogens.
The mentioned uncertainties need to be addressed in order to form a bridge from the current theories of mycoremediation to a practical field trial. Laboratory trials will be the most reliable and cost-effective manner to achieve this. Because the main uncertainties are related to which specific species show promise for application, and to how the known and hitherto unknown species will perform with typical concentrations of contaminants from farm runoff, an experimental method of biological assays (bioassays) seems a sensible way to proceed.
In general, bioassays are used to unveil the effect of a substance on the target organism. Of course, the effect of the contaminants on the fungi is of great interest to us, but the main factor in whether a species shows promise for further practical trials lies in how much it can reduce the concentrations of the contaminating substances in the runoff water. In order to test this, an experimental setup similar to that described in Stamets (2005, p. 61) will need to be used. Artificial runoff channels with a place for mycelium grown on substrate should be constructed in the laboratory. In such a channel, the effectiveness of a species can be tested by introducing water with a known concentration of contaminants ‘upstream’, while the result of the mycofilter is measured from the water quality ‘downstream’. Because such a set-up is most similar to field conditions, it should be the final test to determine which species to include in future field trials.
However, there are many less costly experiments that can be performed to estimate whether a species will perform favorably in the ‘channel experiments’. Singh (2006) describes tests with fungi grown in a liquid medium, and several tests that can be performed on an Agar medium in petri-dishes.
Methods
The research proposal covers three research strategies. There is a initial literature study (phase 1) which is necessary for to identify the fungi which will need to be included in the bioassays regardless of their found presence in the field. The second strategy employed is field work (phase 2) during which the researchers will be required to collect the fungi samples on site. The last research strategy is the experimental strategy (in the form of bioassays) in which the various fungi are exposed to (realistic) concentrations of contaminants.The experiments are divided over three phases (phase 3, 4 and 5). First all samples are included in agar well assays, in which mycelium growth is measured as an indicator of contaminant resistance. The samples that show promises are then included in an artificial channel set-up in which the reduction in the concentration of contaminants can also be measured.
Categories of contamination from agriculture:
- Xenobiotics ( E. Coli, Bacillus spp., Staphylcoccus aureus )
- Pesticides ( anthracenes, pentachlorophenol, alachlor, aldrin, chlordane, DDT, heptachlor, lindane, mirex, atrazine, benomyl - Knapp et al. 2001; Kondo et al. 2003; Gadd 2004 )
- Eutrophication ( Nitrate, N, P, Ammonia )
- Heavy metals ( Cu, Cd, Zn, Pb, Ti, Sr, Bi, Mn, Me, Hg )
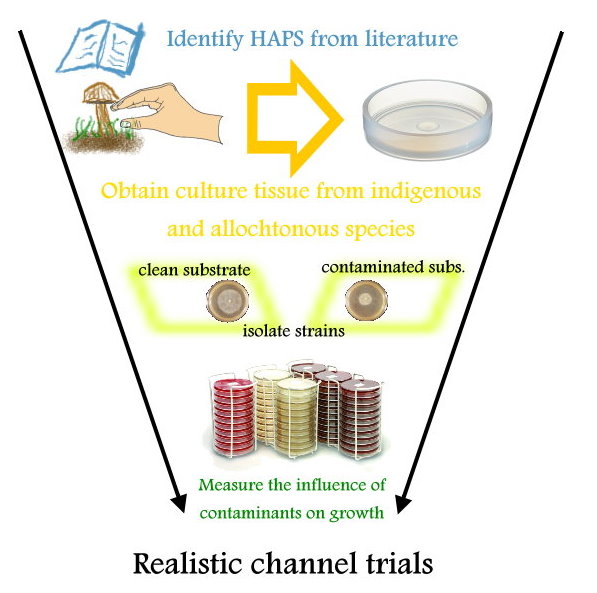
Phase 1: Identify High Application-Potential Species (HAPS) from literature.
A thorough literature study should result in a clear overview of the earlier quantitative data on how fungi species respond to the contaminants that we are interested in. Species that have shown potential to deal with these contaminants should be included in our bioassays even if they are allochtonous to the field sites. Because earlier experiments have shown that this response can differ significantly between strains within a species (Stamets, 2005), additional literature on fungal genetics needs to be reviewed to clarify how large the sample size for the experiments needs to be in order to produce a reliable statistical analysis.The number of contaminants that we propose to investigate is quite large, which could result in experiments that are unnecessarily costly. A review of which contaminants from agriculture cause the most significant societal problems can be used to lower the budgetary requirements.
Phase 2: Collecting tissue samples.
At the broad geographic perspective, sites should be selected from each province of the Netherlands. The literature study will indicate areas of intensive agricultural activity where observed problems with contaminants are largest. The density of selected sites may be larger in the indicated areas. The field work will consist of going to those sites and collecting both samples of fungi (to isolate strains for the lab experiments) and taking water samples from the polluted site.The data collected will enable the researcher to draw correlations between fungi and the contaminated site, resulting in publications on statistical analyses of the natural occurrence of fungi and the agricultural contaminants found in nearby surface waters.
A preliminary strategy for on-site collection can already be formulated, in accord with the guidelines by Stamets (2005). Although a literature review shows that mycorhizzal species can be effectively used for mycoremediation, it has proven challenging to cultivate their mycelium (Jones, 2009). With the applied goal of creating mycofilters in mind, it is more sensible to focus on saprophytic (decomposing) species, as these are easily cultivated on substrates.
Saprophytes require organic litter, shade, and high moisture levels to grow their mycelium and to form mushrooms. In the field they are likely to be found growing in cow dung, under shrubs, and in layers of organic litter.
For the sake of cost, the field work should be planned in mushroom forming seasons, since species identification and subsequent strain isolation from spores or mycelium is a lengthy procedure (Singh, 2006, pp. 10-14).
Phase 3: Isolate strains
After having collected field samples of whole mushrooms there is a need to isolate strains of the various fungi to use in the experimental phases.The technique that will be used is for each individual sample, culture tissue is obtained by carefully removing remaining mycelium (rhizomorphs) from the stem of the collected mushroom. When placed in a petri dish filled with agar substrate, this mycelium will re-enter growth stage (Talbot, 2001; Stamets, 2005). Rhizomorphs are genetically diverse; individual genotypes are to be found in the spores of the mushroom. Therefore, when selecting a strain from culture tissue, the composition of the agar substrate will favor certain genotypes over others.
From each tissue sample, two agar dishes will be grown, the one clean, and the other with low levels of contaminants.
Because the genotype is still diverse in these dishes, the mycelium growth will not occur in a perfect circle when a sample is placed at the center. There will be a ‘leading edge’ which indicates a genotype that is most successful in growing on the particular substrate.
To apply the ‘leading edge’ technique it is necessary to grow the mycelium radially (from the center of the petri dish), but only to observe how large the genetic diversity in the tissue sample is. For the purpose of selecting the ‘leading edge’ for further cultures. New samples are taken from the “leading edge” and placed in the center of fresh agar dishes.
To improve the results the mycelium should be grown on nutrient-poor agar substrate; The mycelium is first grown on nutrient-rich substrate, which is cut in squares, of which one is placed on one half of the nutrient-poor agar. On the other half an uncolonized square of agar (equal in nutrients and size) is placed. This is a better reflection of the natural habitat of mycelium, in which runner hyphae first search for nutrient sources in the direct environment before growing denser mycelium.
This process is iterated until the outgrowth approximates a perfect circle, to indicate that a single strain has been isolated. This technique should also be used in phase 4.
The main purpose of phase 3 is to obtain culture tissue with the least possible genetic diversity for use in the following phases (4 & 5).
Phase 4: Test response to contaminants of isolated strains (agar well assays)
The effect of specific contaminants on the growth of a strain is the primary indicator for the potential of this strain to decompose pesticides, to fix nutrients and metals, and to suppress the growth of xenobiotics. For each collected sample from the field, this phase is entered with two strains from phase 3. A general method to quantify the growth response to a contaminant is called the agar well assay. Within this method there are specific techniques, but for the purpose of this proposal it will be limited to a general overview.There are four main groups of conditions under which the growth of a strain shall be measured.
(1) First is control group consisting of a clean agar substrate.
(2) The second control group consists of a clean agar substrate to which there is a periodic addition of water (by pipetting).
Both of these first 2 groups are used to measure baseline growth against which the other conditions can be compared.
The distinction between the remaining two groups arises from uncertainty in learning effects within a strain. Stamets (2005) describes a technique by which he claims to ‘train’ a strain to secrete novel enzymes and acids in order to deal with a contaminant:
(3) In this condition the agar substrate is clean, and the contaminant is introduced by pipetting a dilution onto the growing mycelium. The contaminant is applied in increasing concentration with resting periods in between.
(4) The fourth condition is more straightforward. The contaminant is added in the preparation of the agar substrate, resulting in a uniform spread across the agar dish.
Within the contaminated conditions (3) and (4), the strains can be tested against single contaminants, all contaminants in a category, all contaminants at once, and realistic mixtures of contaminants, similar to the concentrations found in the collected water samples in phase 2. In order to test for the occurrence of learning effects, samples should not only be exposed to the same concentrations, but also the same total quantity of the substance (Moles).
The exact number of conditions (various concentrations and mixtures) that can be tested against in the set time-frame cannot be predicted. When this phase is performed with the first few strains, a projection can be made of how many times the experiments can run in the set time. At that point the decision can be made to focus on the most important contaminants that were selected in the literature study.
To produce reliable statistical results, it is more important to repeat the same conditions multiple times, than to try the largest possible number of conditions.
This phase will be performed multiple times within the timeframe, each with a limited set of strains. The collected data is of course saved for later publications, but it is also possible to publish on the effects of agricultural contaminants for single species or a set of species, as much of this will be novel work. Based on the results of this phase the strains that show most promise to grow under single- and multi-contaminant conditions are selected for inclusion in the channel trials. If any significant learning effects are observed, it will be interesting to perform the channel trials with the ‘experienced strains’ obtained from this phase 4, in addition to the isolated strains from phase 3.
Phase 5: Semi-realistic channel experiment
The eventual goal of this research proposal is the application of mycofiltration in the Netherlands. The unknown character of the isolated strains will need to be tested in realistically approximated settings. These experiments are more realistic than previously performed trials and experiments which focus on the mycelium and contaminants by incorporating a new aspect, that of water quality. This implies looking at the breakdown of contaminants when the mycelium is contained on a solid substrate and the contaminants are in solution. In previous experiments, either the fungi and contaminants were both in solution, or both in solid form.Creating realistically approximated channel trial means subjecting the different test samples to an artificial polluted environment (water, air) in which the mycelium can interact with bacteria. Saprophytes steer the biological communities creating the first food web in top soil (Stamets, 2005) and thus interact with many of the present organisms.
a. Applying the results in realistically approximated settings:
To mimic outside conditions, the experiments will take place with air as an uncontrolled factor which can contain and transport various micro-organisms. The air should be taken in by means of large windows, as opposed to a ventilation system, for the best approximation.
(1) The mycelium is first grown on a sterile substrate (most probably straw or wood chips).
It is important to consider the influence of substrate choice (e.g. straw, corn stems, wood chips, etc.) on the filtering ability of the colonized substrate. Therefore it is necessary to include a control condition for each substrate that will be used. The occurrence of variation in the results from the control conditions would indicate that the results for strains grown on different substrate should be adjusted or treated as separate groups.
(2) The mycelium has already colonized the entire substrate when it is exposed to the air, the presence of bacteria is not an issue for the research. (3) The mycelium will allow or reject bacteria to co-inhabit the substrate on its own accord.
(4) The only controlled factor throughout this experiment will be focused on the composition of the water (contaminants).
b. Testing contaminant concentrations:
To test the samples in a realistic environment, the maximum level of concentration of a specific contaminant from the water samples from the fields will be taken as the value (c=100%).
A selection of samples from phase 3 (a clean sample and a sample grown from low level of contaminants) and from phase 4 (experienced strains) can then be tested by exposing the samples to the individual contaminants for our realistic environment.
The exposure of the samples will start with contaminant value c=0% (control). The concentration of the contaminant will then be increased by 10% each step. This 10% increase will continue until the concentration of the contaminant is at c=150% (the highest concentration is 50% above that of the highest value of that concentration from the water samples (c=100%)).
After having tested the samples by exposing them to individual contaminants, the samples will be exposed to a mixture of contaminants attempting to recreate the original state of water pollution and mixtures of contaminants which could prove answers to different scenarios on contaminant fluxes.
The dependent variable in this trial is the change in concentration of the contaminants in the water after it has run through the colonized substrate.
This phase will be performed twice with a variety of strains from phase 3 and 4. The strains in phase 3 and 4 will change through the second part of the time frame resulting in the use of different strains the second time the realistic channel tries are done. The data collection from these trials will be saved for publications and if some of the results might prove to be effective, their application into mycofilters would be the next step.
It will be interesting to see whether reaching the desired goal (application of mycofiltration in the Netherlands) will generate interest from various sectors (agricultural, environmental, etc...) if a potent strain is found.
Anticipated Findings
Phase 1:
PollutionWe expect to produce a list of known contaminants which originate from agricultural activity in the Netherlands, favorably ranked by societal relevance. Additionally, it should be clarified which approaches are currently used to deal with these contaminants.
Bio/mycotechnology
The second branch of the literature review is expected to yield a taxonomy of fungal species of which the ability to reduce levels of the mentioned contaminants has been found in earlier controlled experiments. Besides a detailed overview of the relationship between species of fungi and specific contaminants, a record of previous research approaches will be kept.
Mycology
The Nederlandse Mycologische Vereniging provides recent information on species occurrence throughout the Netherlands. From this, an expectation for the findings during the field work will be formed. Literature study should also indicate which sample size is necessary in order for our statistical findings to be representative. The researcher is also expected to take note of laboratory procedures for agar well assays and fungal tissue cultivation, which be found in textbooks such as Talbot (2001).
Phase 2:
From the field work phase we expect to collect samples of fungi and samples of contaminated water. The result should be a diverse and statistically representative number of samples for use in the laboratory experiments.Additional data is recorded during the collection of the samples: date, time, and coordinates. From measurements of the concentrations of contaminants in the water samples, we expect to produce a correlation analysis between species and contaminant occurrence. Mainly for communications purposes, the sites will be plotted on a map of the Netherlands, with species and contaminant occurrence shown in the geographical overview. Date and time are recorded to refine the strategy for later field visits.
Phase 3:
In the first phase of laboratory experiments field samples will be used to create isolated strain tissues of the fungi by in vitro methods. Each field sample (mushroom) will result into two isolated strains of mycelium. One strain will be isolated using contaminants in the substrate and the other strain will be isolated by using a clean substrate.While doing the strain isolation, the growth speed of the fungi will be recorded. These observations cannot yet be used for analysis, since the genetic expression of the mycelium is variable until the isolation is complete. They are merely recorded to check whether the procedure is correct and is being followed correctly.
Phase 4:
The growth response of each strain to each contaminant and mixtures thereof (at various concentrations) is measured and recorded. This is done for two manipulated conditions: contaminated agar, and pipetting a solution of contaminants with increasing concentrations into the dish. Respective control conditions are: clean agar, and pipetting clean water into the dish.The obtained result is a quantitative relation between contaminants and mycelium growth for each strain. This should clarify which strains should be included in the following phase, but also how the growth response is spread within a species.
Phase 5:
The selected strains from phase 4 will be used in ‘channel experiments’ in which their ability to influence contaminants concentrations in flowing water will be recorded (concentrations ‘upstream’ and ‘downstream’ of the myceliated substrate). This will be a direct test of whether strains lower contaminant concentrations significantly enough to justify further field trials. Strains that show significant ability to decompose organic toxins, accumulate metals, fix nutrients, or inhibit the growth of xenobiotics, form a set of candidates which are likely to be used for future application in mycofilters.Possible Conclusions
To present the possible conclusions the relevant question is repeated, followed by a general expectation and its argumentation, and finally a detailed example is given.Question Is there a significant correlation between the occurrence of specific species and specific contaminants?
Expectation We expect to find a significant correlation for a small number of species. Most saphrophytes do not grow on open farmlands, where the levels of contaminants are most similar to those in the surface water. Samples collected near shrubbery at the waterline or from unused agricultural lands may fulfill their water needs from precipitation alone (unrelated to agricultural contaminants), in which case a correlation between species occurrence and contaminants in the surface water is improbable.
Example Analysis of field samples shows that there is a positive correlation between the occurrence of Psilocybe semilanceata and a concentration of phosphates higher than 4 mol/L in nearby surface water.
Question What is the influence of contaminant(s) x1(, x2, x3,...xn) with concentration(s) y1(,y2,...yn) on the growth of strain z.
Expectation Some established properties of fungal genera (Stamets, 2005, pp. 113) can be helpful when forming hypotheses for this category of questioning. Pleurotus species are expected to show no growth impairment when exposed to petrochemicals or xenobiotics. Agaricus species are expected to show no growth impairment when exposed to metals or nitrates. Primary saprophytes are expected to show more growth impairment than secondary saprophytes when nitrates are introduced. Psilocybe species are expected to show a positive growth response when phosphorous-containing contaminants are introduced.
Example In a controlled laboratory setting, Psilocybe semilanceata shows more impaired growth than Stropharia rugoso annulata when exposed to 5 mL solution of Mercury ( 1.2 mol/L ). (comparison between manipulated conditions)
Example In a controlled laboratory setting, Psilocybe semilanceata shows 20% higher growth speed when grown on a substrate containing dihydrogen phosphate (15 mol/L) in comparison to a control condition. (comparison between control and manipulated condition)
Question Which fungi species can reduce the concentration of agricultural contaminants in water?
Expectation We expect to produce a table in which strains are coupled with their efficacy in degrading or accumulating specific contaminant substances, and species means are calculated. In this table some conclusions will be similar to those found in literature, but due to the large number of samples included in this study, it can be expected that at least ten combinations are found that have not been previously reported.
Example A strain of Pleurotus ostreatus has shown to reduce an E. Coli population in flowing water by 80% under controlled conditions.
Recommendations
The conclusions that are drawn from the proposed research will have implications for future mycoremediation strategies. A short recollection from the theory section yields that several distinct strategies are already studied:- changing land management to stimulate the activity of mycorrhizal and saprophytic fungi (De Vries, 2009; Jones, 2009)
- use of liquid-medium fungal cultures in bioreactors (Singh, 2008)
- the incorporation of mycelial mass in the form of mats or sacks in the landscape (Stamets, 2005).
The proposed research has least implications for the first strategy, because ecological factors and soil dynamics have (for a large part) been omitted from the research design. The best that can be hoped for is that the found relationships between species and contaminants can be useful as indicators for suggesting changes in land management.
Implications of our conclusions for bioreactors are not straightforward, as the filtering properties of mycelium differ between liquid and solid cultures (Stamets, 2005). For liquid-medium bioreactors, our results can be used as hypotheses in the research and development of new bioreactors. Also, there might be the potential for a new type of bioreactor design, where mycelium on a solid substrate is used to complement the activity of the organisms in liquid culture.
Our results will be most useful for the third mycoremediation strategy. The current applications of this strategy which are reported have for a great part been based on heuristics (rules-of-thumb). The quantitative relations that are expected as a result of this proposal will help to produce general strategies for species selection. Another straightforward implication is that ecological effects of mycological interventions in a habitat can be studied more specifically for the type of mycofilters that can be used in practice. In the case that the channel set-up proves to be an effective system to reduce contaminant levels in water, this type of system could be used in commercial application quite soon, as expected ecological effects are very limited.
We also have some recommendations for actors outside of the scientific community. If the results show a decrease in water pollution from the application of fungi, this new technology should than be taken as a serious tool for environmental cleanup. The government should look into potential reduction of pollution and create a legislative framework to impose stricter pollution levels. This will invigorate research as the stricter regulation will create an economic incentive in pollution reduction. Biotech companies will be interested in taking this technology further and make it marketable. The cost of production should stay relatively low and most of the investment will go into the research and development to perfect the technology.
The end-users of this new technology (large scale farmers, organic farmers, polluted site managers, etc…) will be the ones who will apply the fruits of this research to care for the land that is in their custody.
References
Berthon, J., Grizard, D. (2004) Process for the treatment of effluents from the food and agriculture industry and industrial effluents, comprising biodigestion by filamentous fungi - Patent EP1464626(A2). Retrieved from http://www.oti.com/oti/patent/20041006-1464626-EP-A2De Vries, F. T. (2009). Soil fungi and nitrogen cycling Causes and consequences of changing fungal biomass in grasslands. Ph.D. Thesis, Wageningen Universiteit.
Deacon J. (2005). Fungal Biology. Cambridge, MA: Blackwell Publishers
Elekes, C. C., Busuioc, G., & I, R. C. (2010). The Mycoremediation of Metals Polluted Soils Using Wild Growing Species of Mushrooms. Engineering Education, pp. 36-39.
Federici, E., Leonardi, V., Giubilei, M. a, Quaratino, D., Spaccapelo, R., DʼAnnibale, A., et al. (2007). Addition of allochthonous fungi to a historically contaminated soil affects both remediation efficiency and bacterial diversity. Applied microbiology and biotechnology, 77(1), 203-11. doi: 10.1007/s00253-007-1143-1.
Gadd, G. M. (2004) Mycotransformation of organic and inorganic substrates. Mycologist 18(2), pp. 60-70
Halberg, N. (2005). Farm level environmental indicators; are they useful?An overview of green accounting systems for European farms. Agriculture, Ecosystems & Environment, 105(1-2), pp. 195-212. doi: 10.1016/j.agee.2004.04.003.
Jones, G. K. (2009). Bioremediation of Contaminated Riparian Zones Using Mycorrhizal Fungi – An Exploration of the Feasibility of Restoration Through Mycoremediation. The Evergreen State College
Knapp, J. S., Vantoch-Wood, E. J., Zhang, F. (2001) Use of wood-rotting fungi for the decolorization of dyes and industrial effluents. In Fungi in Bioremediation. Cambridge University Press, pp. 242-304
Kondo, R., Sakai, K., Wakao, K. (2003) White rot fungi and method for decomposing dioxins using them. Bio Remediation Technologie, Inc. (Japan)
Maanen, J. M. S. V. A. N., Vaan, M. A. J. D. E., Veldstra, A. W. F., & Hendrix, W. P. A. M. (2000). Pesticides and nitratre in groundwater and rainwater in the province of limburg in the Netherlands. Environmental Monitoring and Assessment, pp. 95-114.
Price, M. S., Classen, J. J., & Payne, G. a. (2001). Aspergillus niger absorbs copper and zinc from swine wastewater. Bioresource technology, 77(1), pp. 41-9. Retrieved from http://www.ncbi.nlm.nih.gov/pubmed/11211074.
Schroder, J. (2004). The effects of nutrient losses from agriculture on ground and surface water quality: the position of science in developing indicators for regulation. Environmental Science & Policy, 7(1), pp. 15-23. doi: 10.1016/j.envsci.2003.10.006.
Singh, H. (2006). Mycoremediation : Fungal Bioremediation. Published by John Wiley & Sons, Inc., Hoboken, New Jersey.
Stamets, P. E. (2003) Delivery systems for mycotechnologies, mycofiltration and mycoremediation. Publication number: US 2005/0176583 A1. Retrieved from Google Patents
Stamets, P. E. (2005). Mycelium Running: How Mushrooms Can Help Save the World. Berkeley: Ten Speed Press.
Talbot, N.J. (2001) Molecular and cellular biology of filamentous fungi: a practical approach. Published by Oxford University Press, New York.
Wolf, J., Beusen, a, Groenendijk, P., Kroon, T., Rotter, R., & Vanzeijts, H. (2003). The integrated modeling system STONE for calculating nutrient emissions from agriculture in the Netherlands. Environmental Modelling & Software, 18(7), pp. 597-617. doi: 10.1016/S1364-8152(03)00036-7.
Zwart, D. D. (2005). Ecological Effects of Pesticide Use in The Netherlands : Modeled and Observed Effects in the Field Ditch. Integrated Environmental Assessment and Management, 1(2), pp. 123-134. doi: 10.1897/IEAM.